Organizations
Teaming to Tackle Complex Challenges for the Nation
In our uniquely multidisciplinary culture, we value innovation and routinely push the boundaries of fundamental science. Our program reflects this team approach, bringing experts from different fields in multiple LLNL organizations together to solve complex problems of national importance.

Program Areas
Through four key program areas, the SD organization integrates unique capabilities and diverse expertise across the Laboratory in support of the nation’s deterrence mission:
Weapon Physics and Design
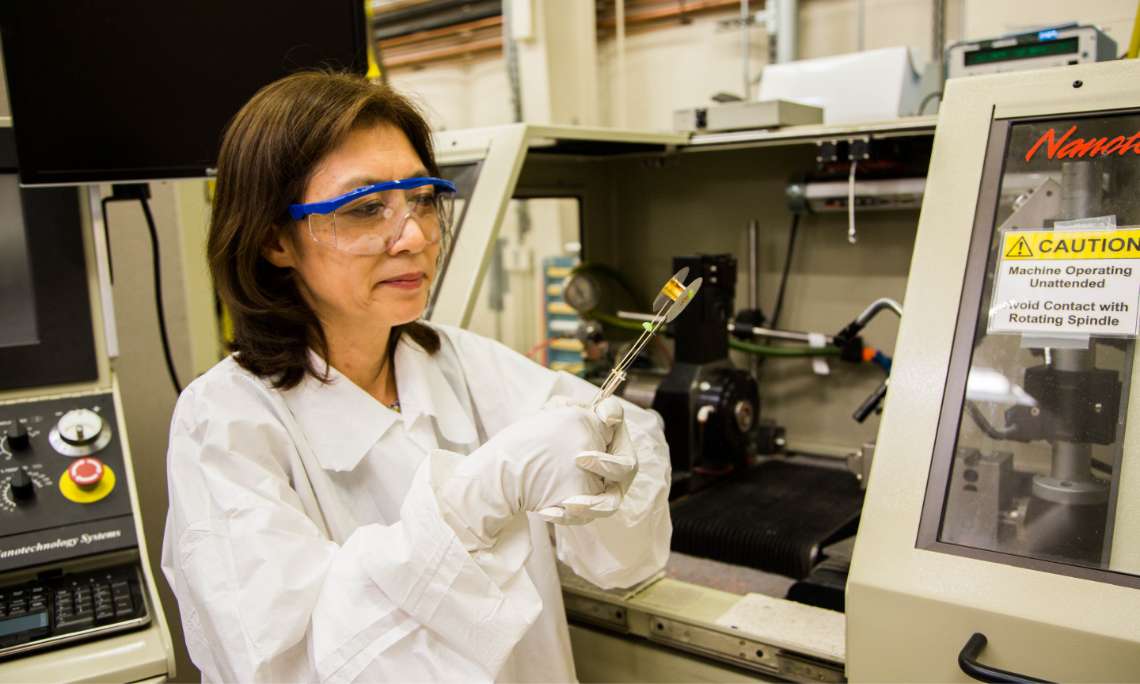
Our multidisciplinary teams are assessing a stockpile that is older than any tested during the underground testing era, while modernizing the physics package of two warheads for deployment on new delivery systems. To do this complex work, we perform applied physics research, developing new models to advance physical and computational sciences relevant to national security missions, and the nuclear stockpile in particular.
The challenge of modernizing stockpile components requires a diverse array of computational and experimental research
- Actinide properties
- Atomic physics
- Nuclear and thermonuclear physics
- Computational physics
- Diagnostics
- Fluid dynamics and turbulence
- Hydrodynamic experiments
- HED physics and experiments
- Material properties under extreme conditions
- Nuclear safety and security
- Plasma physics
- Radiation and particle transport
- Reactive flows and high explosives
- Uncertainty quantification (UQ) and novel statistical methods
- Radiation hydrodynamics
- Laser–plasma interactions
- Implosion physics
- Thermonuclear burn
Weapon Simulation and Computing
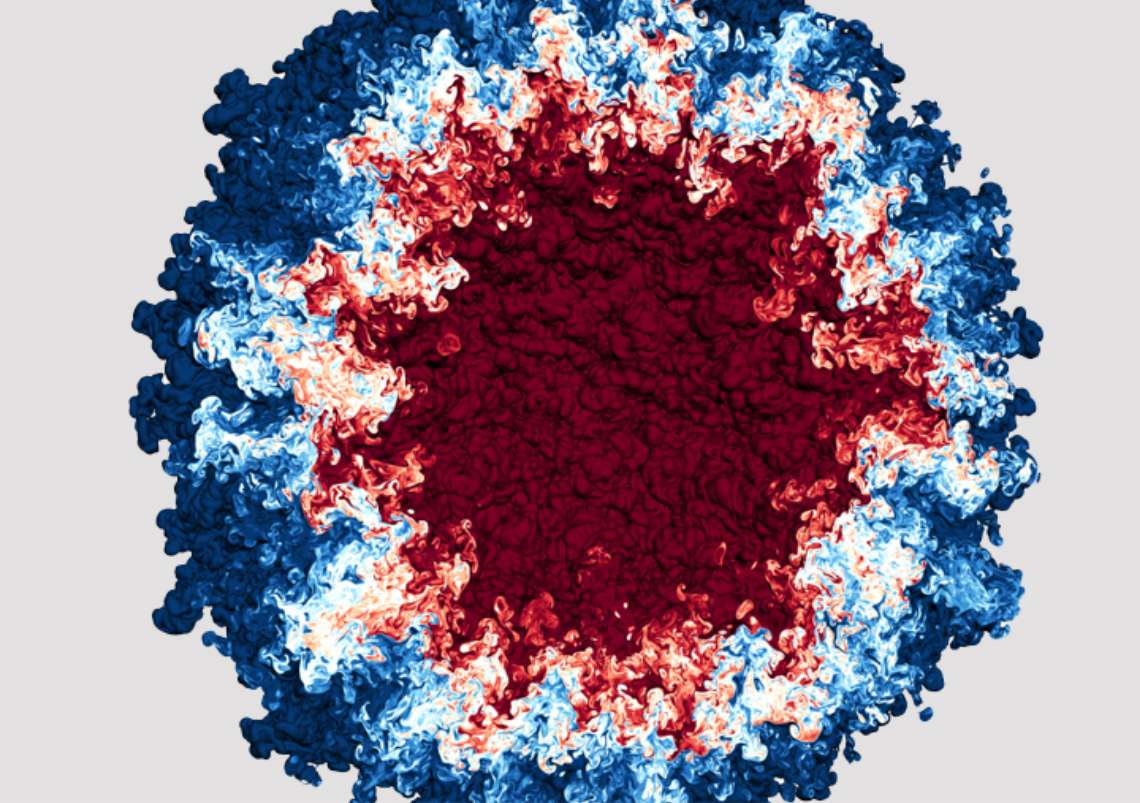
By combining state-of-the-art simulation tools with world-class facilities and computers, Weapon Simulation and Computing (WSC) delivers the capabilities needed to support the nation’s nuclear weapons program as well as the development of inertial confinement fusion and numerous other national security missions. In carrying out these responsibilities, WSC helps advance LLNL’s high-performance computing power.
WSC staff are also active in the open-source community. Other key research areas include extreme computing and hardware architecture.
3D 98-billion-cell hydrodynamic instability simulation (inspired by inertial confinement fusion).
Livermore Computing Complex
WSC meets the challenges of stockpile stewardship at the first-class Livermore Computing (LC) simulation facility, leveraging the systems, tools, and expertise that make these simulations run smoothly and efficiently. LC will be the home of the NNSA’s first exascale system, El Capitan which is expected to provide an over 16x performance improvement on our applications compared to our current flagship system Sierra, which is ranked #6 in the world on the Top500 list.
With a computing floor the size of a football field, LLNL operates one of the world’s largest high-performance computing centers
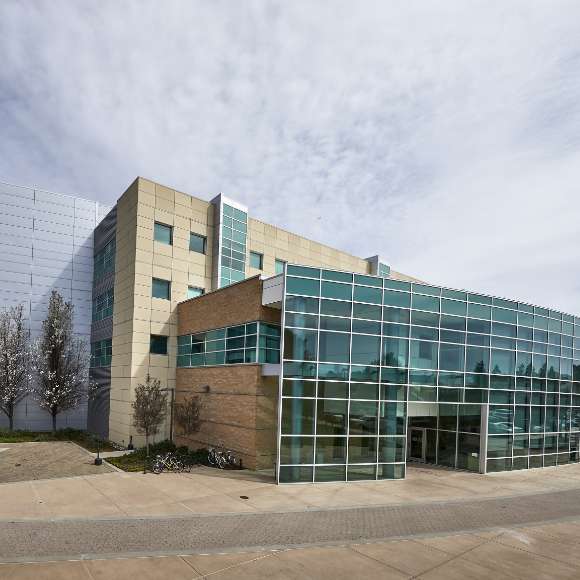
Weapon Technologies and Engineering
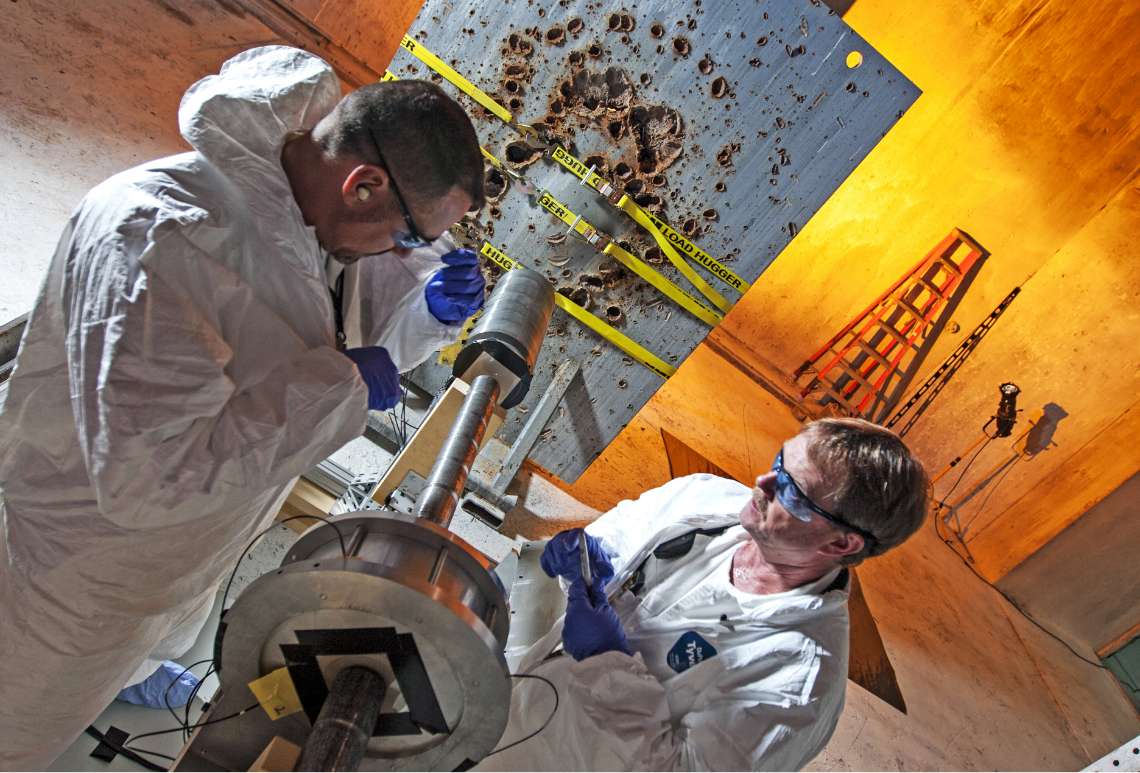
The Weapon Technologies and Engineering (WTE) program is the center of engineering and materials science efforts in support of the weapons program. WTE is responsible for ensuring that physics concepts materialize into realizable designs that reliably meet their military requirements and that our enduring systems continue to remain fit for purpose. WTE’s mission space focuses largely on the nuclear deterrent and also includes end-to-end design and assessment of advanced conventional weapons.
LLNL’s Contained Firing Facility (CFF) can handle up to 60 kg of high explosives and is the largest indoor firing facility in the world.
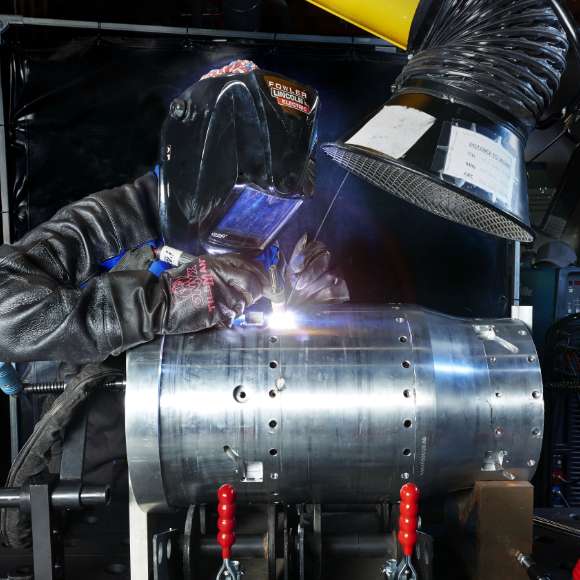
Defense Technologies Engineering Division
Fundamental to the success of the WTE Program are the contributions made by the personnel in the Defense Technologies Engineering Division (DTED) in LLNL's Engineering Directorate, who perform design studies of nuclear warheads and perform leading-edge modeling and analysis of complex engineering problems.
A test unit in support of the U.S. stockpile warhead modernization programs
Weapons Infrastructure
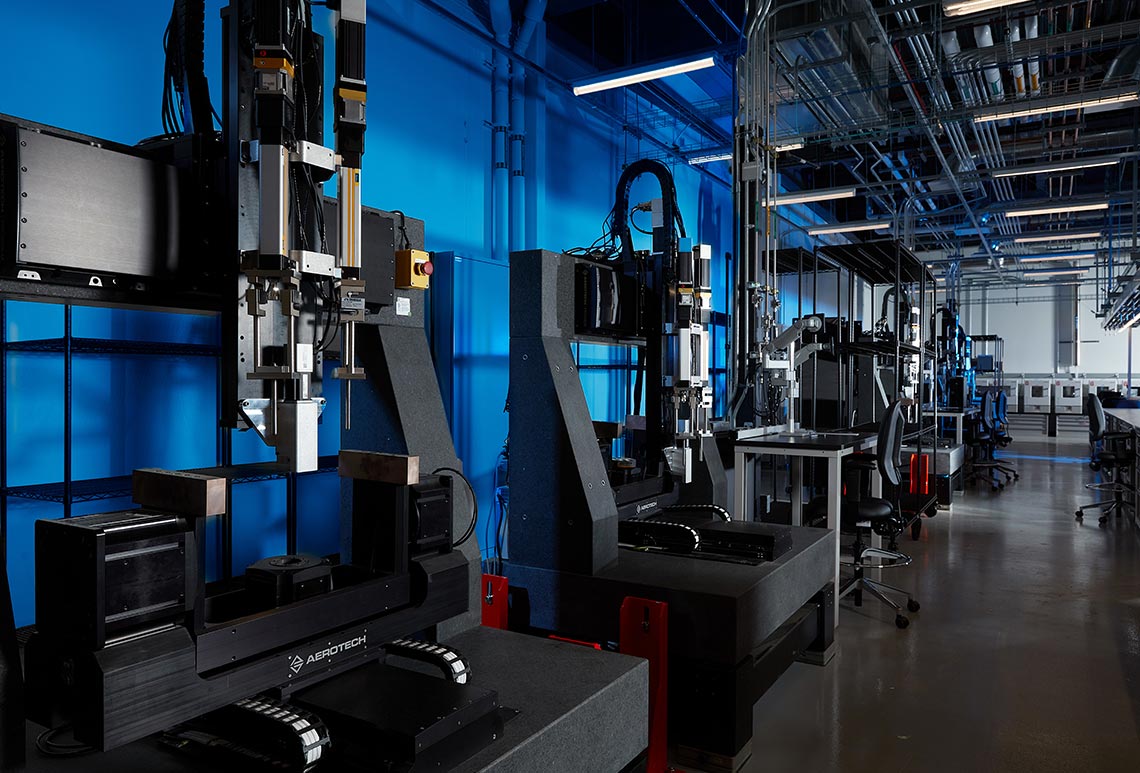
The Weapons Infrastructure Program (WI) delivers nuclear weapon infrastructure capabilities, modernizes programmatic equipment, and performs nuclear weapon enterprise evaluation and planning. These capabilities directly support the execution of NNSA stockpile modernization programs, annual assessment processes, and stockpile S&T and manufacturing modernization.
Special facilities and equipment, such as the Polymer Enclave shown here, are part of our efforts to maintain, upgrade, and modernize mission-delivery infrastructure.
Strategic Partnerships with NNSA and DOD
WI supports NNSA and LLNL decision-making by performing nuclear weapons enterprise modeling for stockpile, infrastructure, and workforce equities at both the Laboratory and Nuclear Security Enterprise scale. WI also manages the LLNL safety, infrastructure, and operations portfolio and serves as the planning, programming, budget, and execution lead with NNSA for the Laboratory’s infrastructure.
Working closely with NNSA, LLNL is realizing cutting-edge infrastructure capabilities that will support stockpile programs and world-class workforce for the Nuclear Security Enterprise.
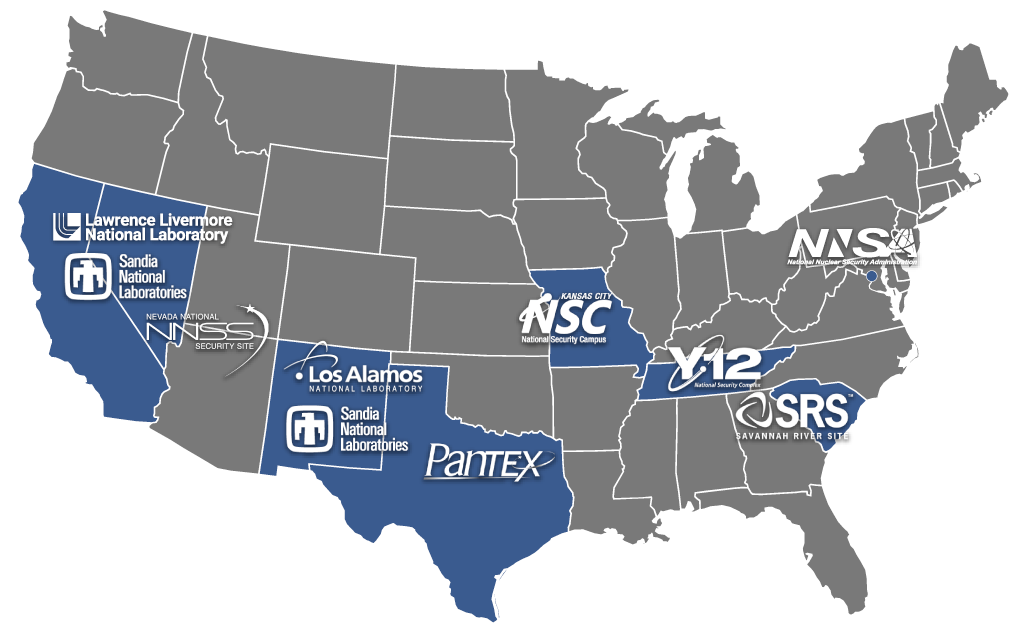
Our multidisciplinary teams are assessing a stockpile that is older than any tested during the underground testing era, while modernizing the physics package of two warheads for deployment on new delivery systems. To do this complex work, we perform applied physics research, developing new models to advance physical and computational sciences relevant to national security missions, and the nuclear stockpile in particular.
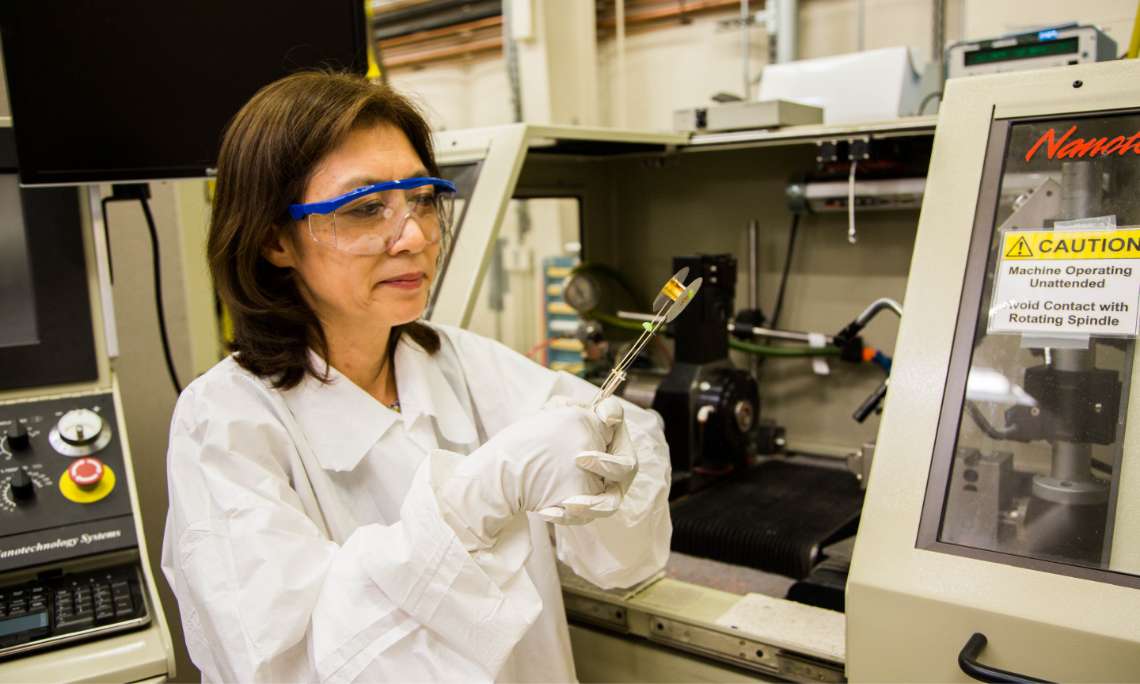
The challenge of modernizing stockpile components requires a diverse array of computational and experimental research
- Actinide properties
- Atomic physics
- Nuclear and thermonuclear physics
- Computational physics
- Diagnostics
- Fluid dynamics and turbulence
- Hydrodynamic experiments
- HED physics and experiments
- Material properties under extreme conditions
- Nuclear safety and security
- Plasma physics
- Radiation and particle transport
- Reactive flows and high explosives
- Uncertainty quantification (UQ) and novel statistical methods
- Radiation hydrodynamics
- Laser–plasma interactions
- Implosion physics
- Thermonuclear burn
By combining state-of-the-art simulation tools with world-class facilities and computers, WSC delivers the capabilities needed to support the nation’s nuclear weapons program as well as the development of inertial confinement fusion and numerous other national security missions. In carrying out these responsibilities, WSC helps advance LLNL’s high-performance computing power.
WSC staff are also active in the open-source community. Other key research areas include extreme computing and hardware architecture.
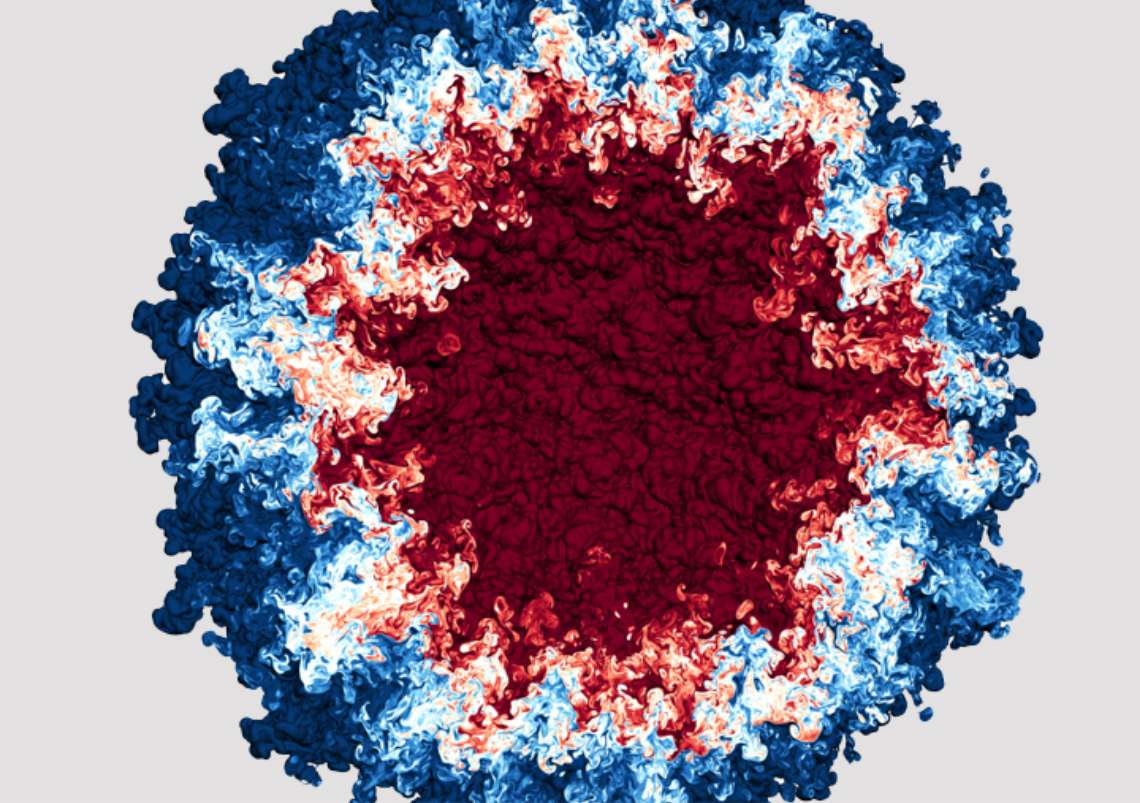
3D 98-billion-cell hydrodynamic instability simulation (inspired by inertial confinement fusion).
Livermore Computing Complex
WSC meets the challenges of stockpile stewardship at the first-class Livermore Computing (LC) simulation facility, leveraging the systems, tools, and expertise that make these simulations run smoothly and efficiently. LC will be the home of the NNSA’s first exascale system, El Capitan which is expected to provide over 16x performance improvement on our applications compared to our current flagship system Sierra ranked #3 in the world on the Top500 list.
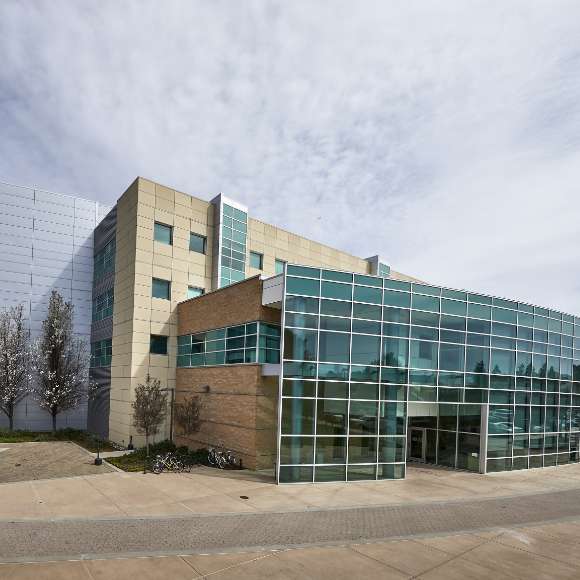
With a computing floor the size of a football field, LLNL operates one of the world’s largest high-performance computing centers
The Weapon Technologies and Engineering (WTE) program is the center of engineering and materials science efforts in support of the weapons program. WTE is responsible for ensuring that physics concepts materialize into realizable designs that reliably meet their military requirements and that our enduring systems continue to remain fit for purpose. WTE’s mission space focuses largely on the nuclear deterrent and also includes end-to-end design and assessment of advanced conventional weapons.
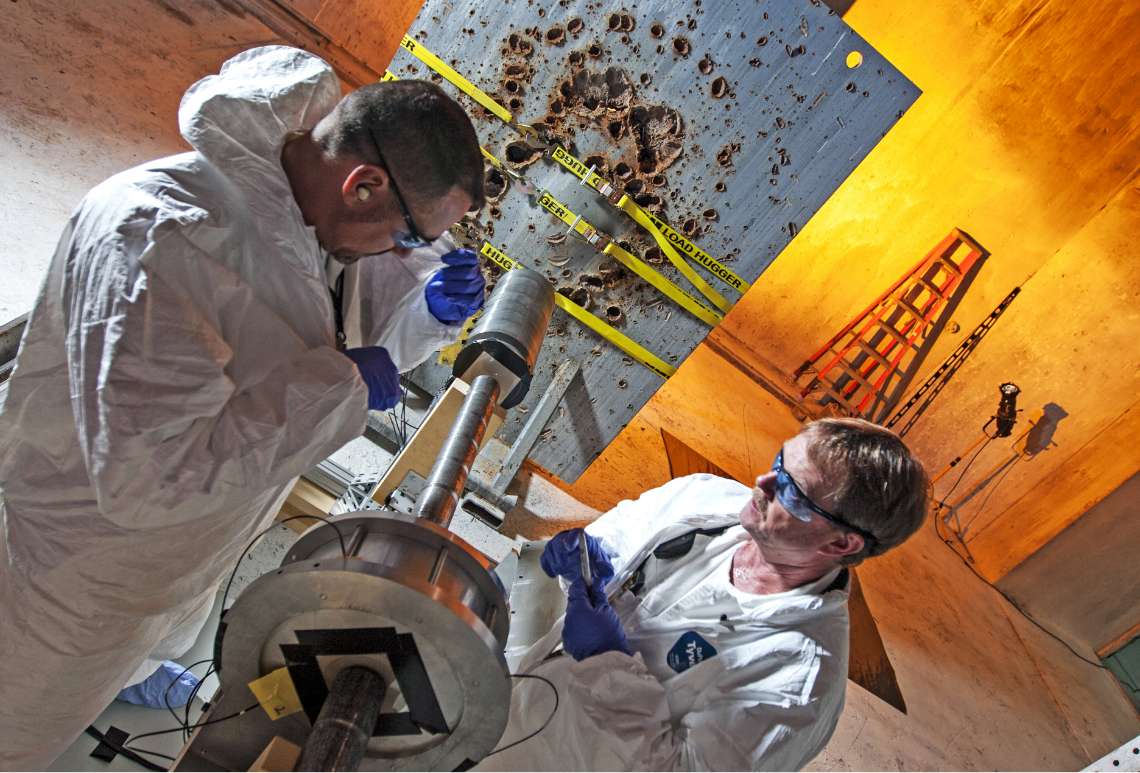
LLNL’s Contained Firing Facility (CFF) can handle up to 60 kg of high explosives and is the largest indoor firing facility in the world.
Defense Technologies Engineering Division
Fundamental to the success of the WTE Program are the contributions made by the personnel in the Defense Technologies Engineering Division (DTED) in LLNL's Engineering Directorate, who perform design studies of nuclear warheads and perform leading-edge modeling and analysis of complex engineering problems.
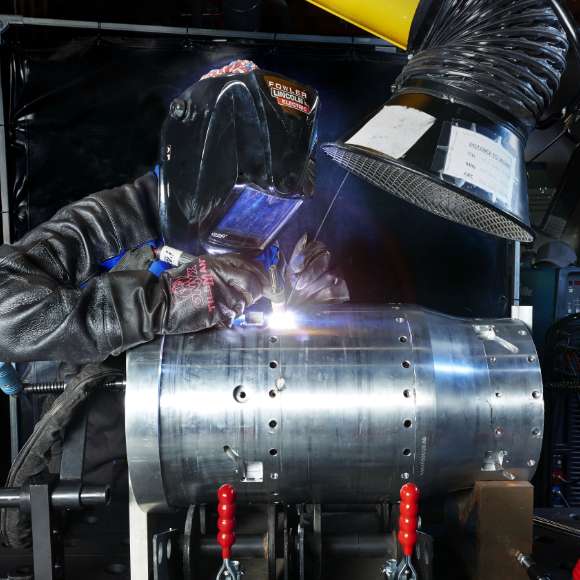
A test unit in support of the U.S. stockpile warhead modernization programs
The Weapons Infrastructure Program (WI) delivers nuclear weapon infrastructure capabilities, modernizes programmatic equipment, and performs nuclear weapon enterprise evaluation and planning. These capabilities directly support the execution of NNSA stockpile modernization programs, annual assessment processes, and stockpile S&T and manufacturing modernization.
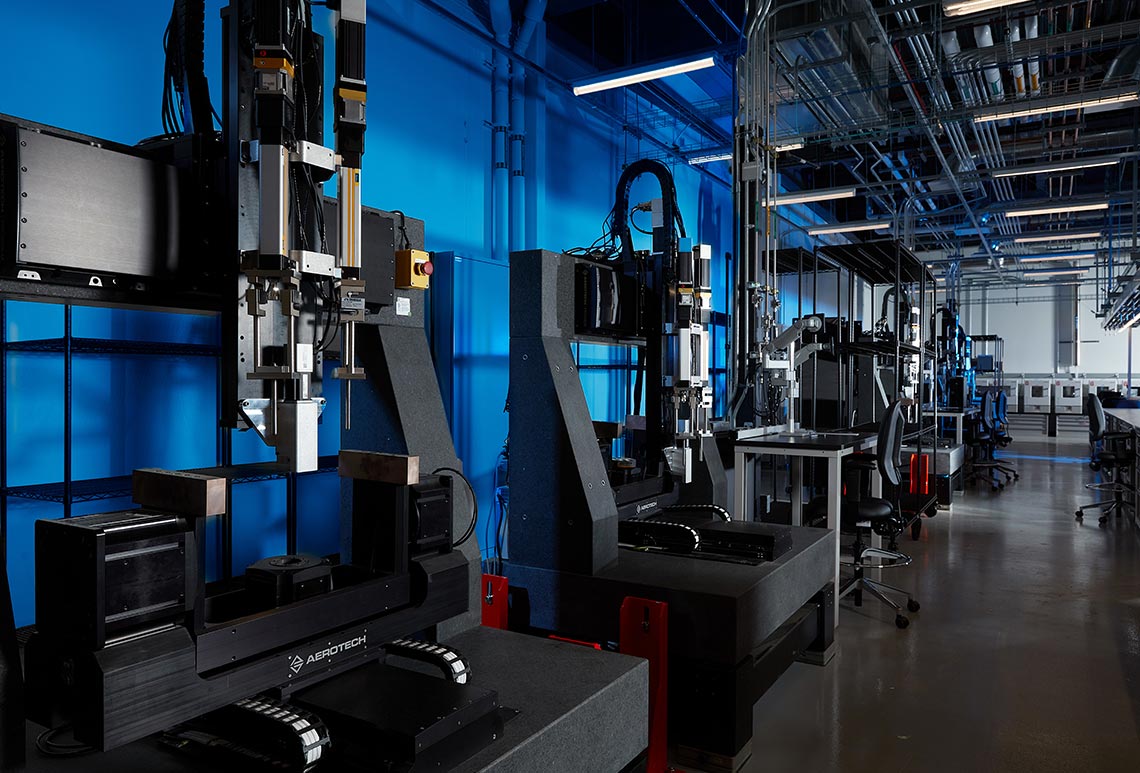
Special facilities and equipment, such as the polymer enclave shown here, are part of our efforts to maintain, upgrade, and modernize mission-delivery infrastructure.
Strategic Partnerships with NNSA and DOD
WI supports NNSA and LLNL decision-making by performing nuclear weapons enterprise modeling for stockpile, infrastructure, and workforce equities at both the Laboratory and Nuclear Security Enterprise scale. WI also manages the LLNL Safety, Infrastructure, and Operations portfolio and serves as the Planning, Programming, Budget, and Execution lead with NNSA for the Laboratory’s infrastructure.
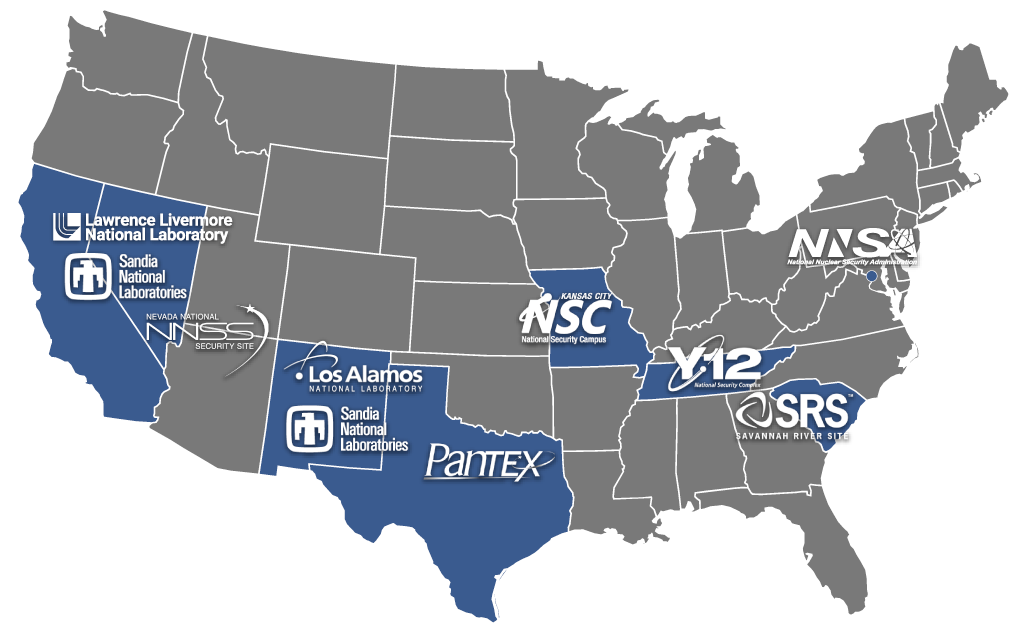
Working closely with NNSA, LLNL is realizing cutting-edge infrastructure capabilities that will support stockpile programs and world-class workforce for the Nuclear Security Enterprise.

Enabling Organizations
World-changing science and engineering doesn’t happen in a vacuum. Operational and technical experts within the weapons program are the key to keeping the doors open and gears turning. These experts specialize in facility management, IT applications and infrastructure, administrative support, business systems analysis, interfacing with collaborators in the UK, assuring the quality and safety of research activities, and safeguarding sensitive information.
To staff these key operational activities, we have three divisions within the weapons program focused on empowering operations, administrative, and weapon physics staff to achieve their potential. Experts from these divisions team closely with staff from four discipline organizations within LLNL to support our mission of modernizing the U.S. nuclear stockpile.
Multidisciplinary Organizations